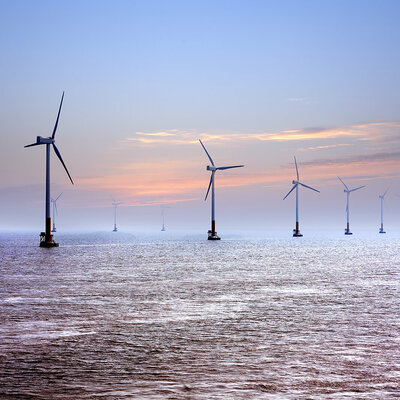
What is Flexibility?
Flexibility in the power system refers to the ability to adjust to changes in electricity supply and demand. It is crucial for integrating intermittent distributed energy resources (DER) like wind and solar, which fluctuate based on weather conditions. A flexible grid can also accommodate demand variations, store excess energy, and respond to system disturbances or outages. This adaptability helps lower costs, improve efficiency, and maintain grid stability. Flexibility is key to transitioning to a cleaner, more resilient, and sustainable energy system.
TSO-DSO Coordination
With the increased flexibility in distribution networks, the coordination between Transmission System Operators (TSOs) and Distribution System Operators (DSOs) becomes essential to efficiently manage grid flexibility. This coordination ensures that both transmission and distribution networks can leverage DERs while maintaining grid stability and addressing operational challenges like congestion and balancing. There are three primary coordination models: centralised, decentralised, and hybrid.
Centralised Coordination Model
In the centralised model, the TSO assumes full control over dispatch decisions for resources in both transmission and distribution networks. Here, the DSO’s role is limited to providing the TSO with relevant operational data, which the TSO uses to optimise and dispatch assets across the grid. This model simplifies coordination since a single central entity, typically the TSO, is responsible for balancing and stability. However, the TSO’s optimisation problem becomes more complex as it must consider both transmission and distribution constraints, leading to potential computational difficulties in large-scale networks.
Decentralised Coordination Model
In the decentralised model, each DSO is responsible for managing resources within its own distribution network and coordinates with the TSO only by sharing aggregated flexibility information. The DSO has more autonomy to resolve local issues, making this model well-suited for systems with high levels of DERs and distributed control. Since the TSO does not require detailed operational data from the DSOs, the volume of data transfer is reduced, lowering communication overhead and potentially improving data privacy. However, this model can create challenges for broader grid stability as DSOs may have varying operational priorities that could conflict with those of the TSO, complicating system-wide optimisation.
Hybrid Coordination Model
The hybrid coordination model combines elements of both centralised and decentralised approaches. In this model, the TSO is still responsible for grid-wide optimisation, but DSOs validate and aggregate flexibility bids from resources within their networks. The validated bids are then shared with the TSO, who includes them in the larger optimisation problem. This method distributes some responsibilities to the DSO level, reducing the computational burden for the TSO and mitigating data transfer requirements. The hybrid model also allows each DSO to manage local constraints, while still ensuring that the flexibility resources contribute to overall grid stability under TSO oversight. Although this model improves efficiency and adaptability, it requires robust communication protocols and may involve complex decision-making processes at both the DSO and TSO levels.
Aggregation Methods for Flexibility
To facilitate these coordination models, aggregation methods for flexibility resources are crucial. DERs and Flexible Power Units can be aggregated using geometric, Data driven and optimisation-based methods, where DSOs combine the flexibility potential of local resources into an aggregated offering. These methods ensure that flexibility resources are utilised efficiently and can be deployed in a way that respects local constraints while supporting the broader needs of the transmission system.
Market and Grid Coordination
Market-based flexibility and grid-based flexibility are complementary approaches that can be coordinated to enhance the overall efficiency and reliability of the power system. Market-based flexibility relies on economic incentives, where market participants—such as power plant operators, storage operators, and demand response providers—are paid to adjust their output or consumption in response to price signals or system needs. This allows for the dynamic allocation of resources, ensuring that the most cost-effective flexibility options are deployed first. Grid-based flexibility, on the other hand, involves the operational capability of the grid to balance supply and demand within power system constraints, often through technical solutions such as reserves, interconnections, and the use of storage.
Coordinating these two forms of flexibility involves aligning market signals with grid operations to ensure that flexibility resources are used optimally. For instance, grid operators can forecast demand and generation variability, and then signal the market to activate certain resources, such as flexible generators or demand-side response, when needed. In turn, market participants can offer their services through flexible pricing mechanisms, allowing grid operators to select the most appropriate resources based on cost and availability. Moreover, grid information indicating peak times can be integrated into market mechanisms, allowing them to respond to price signals and provide services like peak shaving.
A coordinated approach helps avoid over-reliance on any single resource type, ensuring a more resilient and efficient system. It also encourages greater participation from a wide range of players, from large utilities to smaller, decentralised energy producers. To support this coordination, robust data exchange and communication platforms are essential, enabling real-time visibility and responsiveness between market participants and grid operators. Additionally, regulatory frameworks need to incentivise both grid-based and market-based flexibility, ensuring that the cost of providing these services is shared equitably. Ultimately, effective coordination ensures that the power system can meet demand reliably, efficiently, and at the lowest possible cost while facilitating the transition to a cleaner, more sustainable energy future.
Redispatch 3.0
Redispatch 3.0 project aims to improve the exchange of information between DSOs and TSOs by advancing an existing congestion management Redispatch 2.0 framework. In this project, our research group setup hardware-in- the-loop simulation environment for validating distribution system control applications. This experiment setup represents real-world power systems with key market players such as TSO, DSO, grid operators the meter operator, aggregator, and the customer. The experiment setup includes both physical and virtual devices to replicate realistic environment for testing and validation such as remote terminal unit, energy management system, smart meter gateway, controllable local system (CLS). Several use cases can be tested and validated in real-time manner such as congestion management at low-voltage system, aggregation approaches for coordinating flexibilities.
International Smart Grid Action Network (ISGAN)
Aggregators can enhance the number of active customers and unlock untapped DERs in low-voltage systems by serving as intermediaries to manage and coordinate flexibility among market players. Our research group investigates coordination strategies for TSO-DSO-Customer interactions. We have presented a discussion paper titled “How Can Aggregators Improve TSO-DSO-Customer Coordination in Digitalized Power Systems?” This paper examines the roles and definitions of aggregators and outlines potential coordination approaches among aggregators, grid operators, active customers, and other market participants, utilizing the Smart Grid Architecture Model (SGAM).
E-Mail: sharaf.aldin.alsharif(at)offis.de, Phone: +49 441 9722-748, Room: Flx-E
E-Mail: jirapa.kamsamrong(at)offis.de, Phone: +49 441 9722-233, Room: E85
Matteo Barsanti and Jan Sören Schwarz and Faten Ghali and Selin Yilmaz and Sebastian Lehnhoff and Claudia R. Binder; Energy Research & Social Science; 01 / 2025
Otte, Marcel and Kamsamrong, Jirapa and Lehnhoff, Sebastian; ISGAN 2024; July / 2024
Schwarz, Jan Sören and Perez, Leonard Enrique Ramos and Pham, Minh Cong and Heussen, Kai and Tran, Quoc Tuan; 2024 Open Source Modelling and Simulation of Energy Systems (OSMSES); 09 / 2024
Clausen, Christian Skafte Beck and Lehnhoff, Sebastian and Schwarz, Jan Sören and Jørgensen, Bo Nørregaard and Ma, Zheng Grace; Energy Informatics; 2024
Pirta-Dreimane, Rūta, Andrejs Romanovs, Jana Bikovska, Jānis Pekša, Tero Vartiainen, Maria Valliou, Jirapa Kamsamrong, and Bahaa Eltahawy; Energies; April / 2024
Otte, Marcel and Krüger, Carsten and Das, Pratyush and Rohjans, Sebastian and Lehnhoff, Sebastian; Energy Informatics Academy Conference; October / 2024
Otte, Marcel; DACH+ Conference on Energy Informatics 2024; October / 2024
Otte, Marcel and Krüger, Carsten and Das, Pratyush and Rohjans, Sebastian and Lehnhoff, Sebastian; DACH+ Conference on Energy Informatics 2024; October / 2024
Schwarz, Jan Soeren and Pham, Minh Cong and Tran, Quoc Tuan and Heussen, Kai; 2023 Asia Meeting on Environment and Electrical Engineering (EEE-AM); 01 / 2024
Raczka, Sebastian and Puhe, Frederik and Krueger, Carsten and Arph, Jan and Rehtanz, Christian; ETG Congress 2023; Juli / 2023